
Humans, or at least the first members of our biological family, first arrived in Europe at least 500,000 years ago. Since the last Ice Age officially ended only about 10,000 years ago, most of European prehistory took place against the backdrop of Ice Age climates, and much of Ice Age archaeology consists of understanding these climates and their effects on the lives of early Europeans. These effects must have been varied, because the climates themselves were varied.
We tend to think of the Ice Ages (or the Pleistocene, the geologically correct term for the height of the Ice Ages) as a time of bitter cold, a time long past. The Ice Ages, which began over 13 million years ago when the great Antarctic ice sheet formed, officially ended when the last of the great ice sheets retreated toward the poles or into the mountains almost 10,000 years ago (Fig. 1). Nevertheless, the Ice Ages have almost surely not ended.
Nor were the Ice Ages a time of unvarying cold. They were instead marked by great swings in the world’s climate, from frigid to much warmer than today. London, for example, is known for its fogs and chilly damp, yet in the very midst of the “Ice” Ages, hippopotami wallowed in the Thames. What is more, the present warm spell is still young, as geologists reckon time. Europe saw its first major glaciers over 3 million years ago. During these 3 million years, in between the cold times, there have been many warm spells that lasted longer than today’s. It is only because our lives are short that we have no memory, even in history, of anything but warmth; and it is for this reason that we think of warmth as permanent instead of transitory. Though we ourselves were born in the midst of a warm spell, it will not last.
What Caused the Ice Ages?
If we consider the mechanism that caused world glaciation, we can see why it is likely that the great glaciers will return. The most probable explanations involve forces so huge as to dwarf even the greenhouse effect, and are of a kind that are likely to reoccur. Furthermore, whatever the mechanism, it will inevitably trigger a snowball effect. Most of the earth’s warmth comes from the sun. Glaciers, being white, reflect sunlight; as they grow, the amount of sunlight reflected increases. As a consequence, temperatures fall and the glaciers grow at an even faster pace. The opposite occurs when they begin to shrink. Less light is reflected, more heat is trapped, and the glaciers retreat ever more rapidly.
The most commonly accepted explanation for the Ice Ages is a hypothesis named for its main elaborator, Milton Milankovitch. This hypothesis invokes several cyclical changes in the relationship between earth and sun (Fig. 2). First, the angle of the earth’s axis of rotation increases and decreases over a period of 41,000 years. This angle is responsible for the changing length of night and day from summer to winter. The fact that it increases and decreases means that sunlight received at different latitudes varies though the 41,000-year cycle.
Second, the earth’s orbit also changes cyclically every 100,000 years. This orbit is not circular but elliptical, so that the distance between sun and earth varies during the year. The gravitational pull of other planets alters the elongation of this ellipse. When, as now, the orbit is close to circular, average distance from the sun is low, and annual solar radiation is high, but the orbit is elliptical, the earth spends part of the year farther from the sun than normal, and receives less heat.
Third, the point along the earth’s orbit at which the solstices or equinoxes occur varies through two superimposed cycles of 19,000 and 23,000 years. If the orbit were circular, this variation would make no difference, but since it is elliptical, this means that sometimes the (northern hemisphere’s) long summer days will fall near the sun, while at other times they will occur far from the sun. (For a fuller discussion of this theory, see Imbrie and Imbrie 1979.)
Although most scholars accept the solar explanation, there are some who disagree. For example, it has been argued that periods of massive volcanic activity could have reduced sunlight enough to trigger the feedback between glaciation and reflection of sunlight described above. It has even been suggested that climatic change is not orderly but random. If this is so (and it is true that fluctuations in weather are greater from decade to decade than from year to year), than the Ice Ages have no explanation; they happened by chance alone. For scientists, however, such a hypothesis is not terribly satisfactory. By its very nature, it can never be disproven—and the possibility of disproof is what makes a hypothesis scientific.
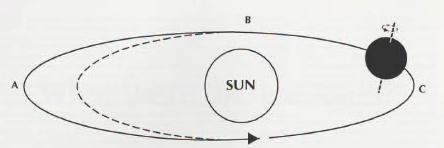
The Geological Legacy of the Ice Ages
The geological effects of glaciers are twofold: erosion and deposition of eroded material. As the ice flows—and slips and grinds—across the underlying bedrock, it leaves telltale traces. The rock is often polished and grooved. Under continental ice sheets (see Fig. 1), whole regions can bear witness to such treatment. Most of Canada and large parts of the United States today consist of great expanses of smooth, flattened rocky landscapes where the great North American ice sheets spared few hills and little soil, and numerous lakes fill the shallow depressions scoured out by the passing ice. The effects of mountain glaciers are more restricted in scope, but more spectacular in terms of scenery (Fig. 3).

Glacial deposits, too, are characteristic. At the point where a glacier finally terminates, it dumps the material it has eroded. This material, called “till,” will mark the presence of a glacier for future geologists (Fig. 4). When this point is stable—when the end of a mountain glacier or the periphery of an ice sheet does not move for a long time—till is deposited in ridges called “moraines.” When the ice has disappeared, these moraines remain behind, drawing a map of the vanished glacier on the modern landscape.
Glaciers are also responsible for a windblown sediment called loess. Much of the enormous quantity of rock that glaciers erode is reduced to a very fine powder called rock flour. The meltwater of glaciers is often called glacial milk, because it runs white with its load of rock flour. In glacial times, cold and aridity discourage vegetation near the margins of the continental ice sheets, so that rock flour deposited by glacier-fed streams is poorly protected. Strong winds pick it up in huge quantities, depositing it downwind in thick blankets. These wind-deposited sediments provide the fertile soil that covers vast areas of the northern hemisphere (Fig. 5).
Of course, glaciers are not the only markers of cold times. Changes in climate are also reflected in the geological record by variations in erosion, deposition, and weathering (Fig. 7). Precipitation also changed during the course of the Ice Ages, and it too affected the geological record.
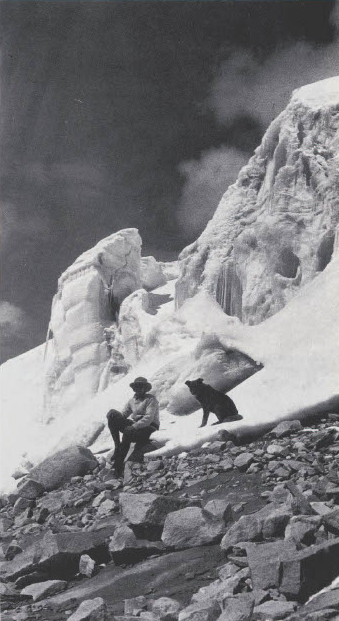
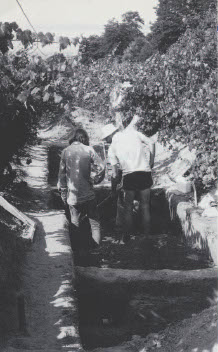
Frost, for example, leaves many traces. Caves are of special interest to archaeologists, for they are often rich in artifacts. The natural fill in which artifacts are found comes in large part from cave roofs. Much of this rock is pried off when water enters cracks, Freezes, then expands. The colder the climate, the deeper this freezing goes, and the larger the chunks of rock pried loose.
Climate also determines the rate of chemical activity. Since heat and humidity are conducive to the breakdown and alteration of rock and sediment, warm periods are often indicated by heavily altered sediments. Geologists call such chemically altered horizons “soils,” and can recognize those produced in different environments—in grasslands as opposed to forests, for example.
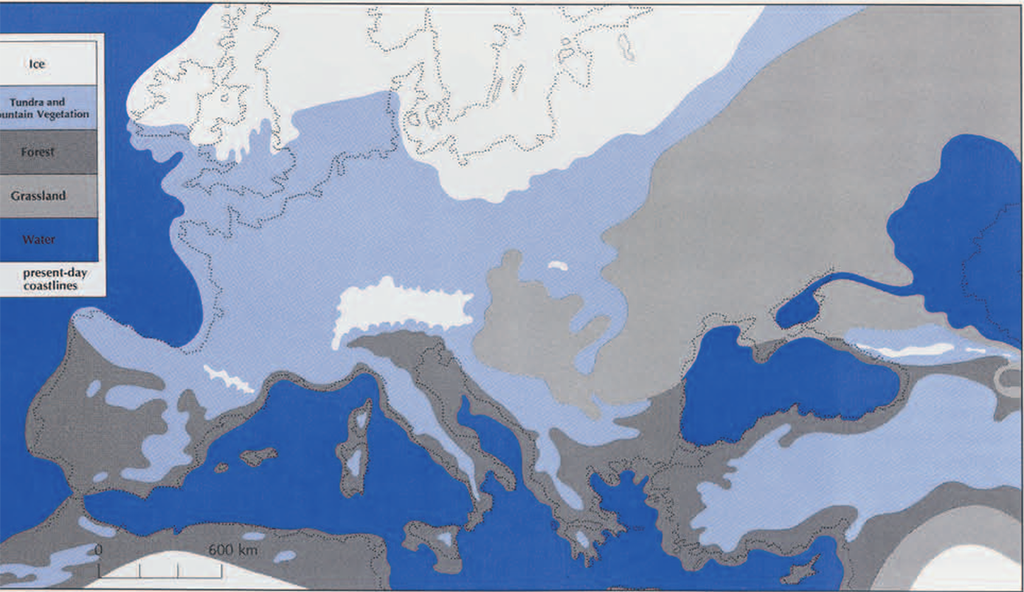
The glaciers had their most far-flung effect not on land hut in the oceans. The water they locked up as ice had to come from somewhere, and that was, ultimately, from the oceans. The glaciers were often so huge that sea levels were much lower than today (Fig. 6). Land that is presently inundated was, in glacial periods, dry. Twenty thousand years ago Britain was connected by a great plain to France and Denmark. But when the climate was warmer and the glaciers smaller than today, the oceans were deeper. Ancient beaches, complete with sea shells, are found far above today’s shorelines (Fig. 8).

Rising and falling sea levels caused changes far inland as well. Rivers would alternately fill and erode their valleys, even far upstream, as their mouths rose and fell through the millennia. Since rivers also tend to move from side to side, such cycles of building and destruction created terraces, step-like features roughly parallelling the rivers. Terrace sequences can be quite complicated, and sometimes almost impossible for the non-geologist to recognize, but this did not keep one single-minded French archaeologist from telling an unfortunate cab driver to “turn left at the third terrace.”
Plants and animals must respond to climatic change, and both leave traces behind them. Sufferers from hay fever will be discouraged but not surprised to learn that pollen is remarkably resistant to the ravages of time. Pollen specialists, or palynologists, recover fossil pollen from archaeological sites by soaking dirt in acid strong enough to destroy it, but not strong enough to damage the pollen grains, which under a microscope are almost as recognizable as the plants which they come (Fig. 12). Thus palynologists can count grains rom different species in a sample of fossil pollen and reconstruct the vegetation of ancient landscapes. The job in not simple, though. Some kinds of pollen can travel hundreds of miles on the wind, and palynologists endlessly debate the theoretical niceties involved. Still, pollen is one of the most useful clues to climate in areas not directly affected by glaciers.
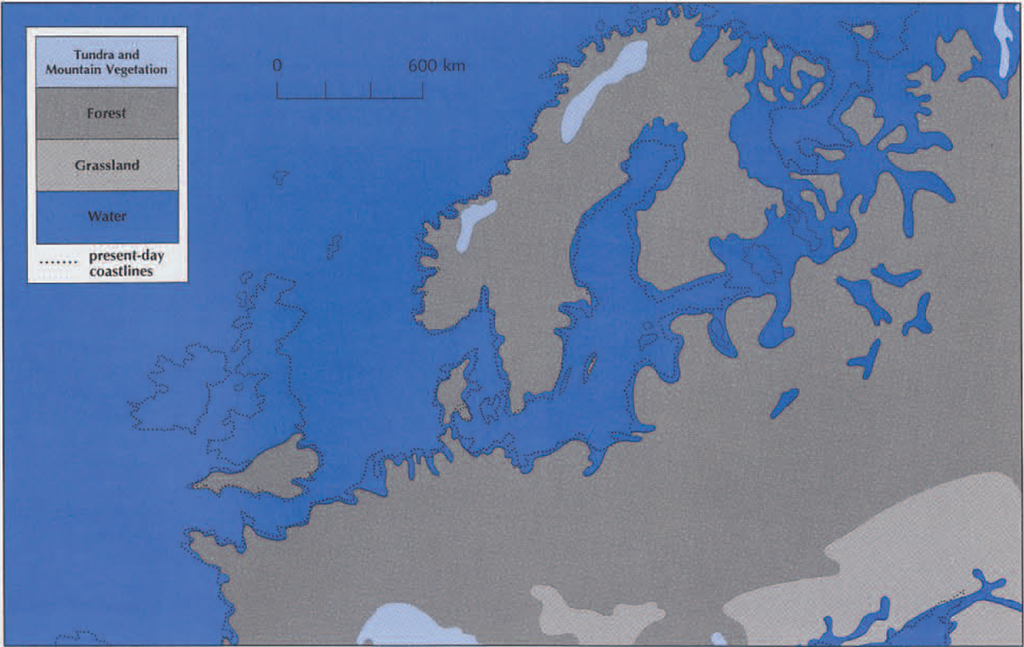
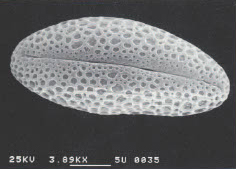
Animals, too, respond to their environment, and their bones survive almost as well as pollen. If the bones a paleontologist finds belong to a species that is still alive today, they provide a clue to ancient climatic conditions. Knowing the environmental preferences of the living species, the paleontologist assumes that their Ice Age counterparts inhabited a similar environment. In southern France, during several periods, people hunted reindeer more than any other animal. Obviously, the climate then would not have attracted sunbathers to the Cote d’Azur. Yet even long extinct species can provide information about climate. Woolly mammoths and woolly rhinoceri have been dead for millennia, yet we know they belonged in a climate far colder than that of their living relatives. For one thing, we know they had warm coats of hair because frozen carcasses have been found with the hair intact. (Pictures painted by prehistoric artists also depict this hair very clearly.) Anatomy provides another clue. The teeth of woolly mammoths are specialized for grazing. For this reason, and because they are normally found together with the remains of another grazer, the horse, it is probably safe to say that they lived on grasslands or steppes. and the extent of prehistoric grasslands can be judged in part by where their bones are found.
Dating the Ice Ages
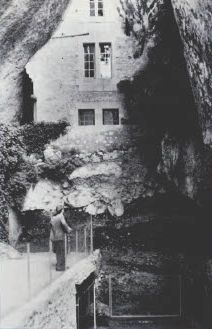
The foundation of all geological dating is stratigraphy, the analysis of the layering of rocks or sediments. Since various factors determine the appearance of a sediment—where it comes from, whether it was eroded by ice or by water, whether it was deposited by wind or water, how much it has been weathered—changes in sediment reflect changes in environment. When archaeologists dig a very old site, they are usually digging through a series of layers representing such changes (Fig. 9). bn principle, the oldest layers are at the bottom, the youngest ones on top. By keeping track of the layers where artifacts are found, an archaeologist should be able to determine at least the relative ages of these finds.
In actual practice, stratigraphy can become quite complicated. For one thing, sediments laid down today are likely to be eroded tomorrow, so that most sites are plagued by serious stratigraphic gaps. For another, layers can be time-transgressive: the same layer can be older in one place than in another. bf the point at which a glacier terminates and deposits till is stable, a moraine is formed; but if the tip of the glacier is retreating as the climate gradually warms, it will lay down not a ridge but a continuous sheet of till that will be oldest at the lower end and centuries or millennia younger at its upper end. Finally, in places such as caves and stream beds, sediments are not laid down in simple layer-cake fashion, but in a much more complicated, even chaotic, manner (Fig. 11).
There is another problem with stratigraphy. Although it is useful to re-create the history of deposits in one excavation, archaeologists also need to know, in many cases, which of two sites is older. If they happen to be close to one another, the same layers of sediment may be found in both, and a geologist may be able to recognize them. Since this is rarely the case, geologists must instead look at each layer at each site, try to determine the conditions under which it formed, and then to correlate them using arguments such as, ‘The cold spell represented by layers A and Upper B at site X is the same cold spell represented by layers 4b through 8 at site Y.’ Needless to say, this is a time-consuming and complicated process.
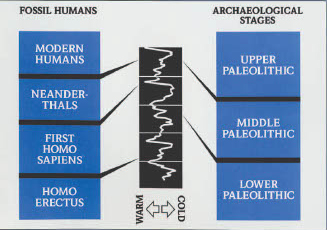
During the last few decades, geologists have found a remarkable record of the world’s climate preserved under the deep oceans. In places underwater where erosion does not occur, geologists can drill through the sediments, recovering cores containing unbroken stratigraphic records. Fortunately, in such sediments they also find a good measure of climate, the shells of plankton called Foraminifera. Like other animals, species of Foraminifera differ in their climatic preferences, so a scientist with the patience to count them can determine the temperature of the water in which they lived. A more subtle but perhaps more accurate way to use these tiny animals is to measure the ratio of two isotopes of oxygen, oxygen-16 and oxygen-18, found in their shells, for this ratio is a good measure of the amount of water that was locked up as glacial ice at the time the animals lived.
We do not have deep sea cores going back more than a few hundred thousand years, but for this time period at least, they give a remarkably complete picture of variations in the world’s climate (Fig. 10). They thus provide a scale to which geologists can try to fit their terrestrial stratigraphies. This is done by matching cold spell to cold spell and warm spell to warm spell. Unfortunately, there are so many of both that it is often not clear which one goes with which. Fortunately, geologists can get help from other dating methods.

The techniques described so far give only relative ages; they let us know only whether one layer is younger, older, or of the same age as another. However, methods are available that give absolute ages, expressed in years before the present. Those that can be used on Ice Age materials are for the most part based on radioactive decay. Not to be confused with organic decay, radioactive decay involves the transformation of an unstable, radioactive substance into less radioactive, more stable substances. Because it proceeds at a very regular rate, it can be used to measure time—if we can find a material that provides a known starting point. Organic substances such as bone or charcoal are one such material, since the amount of radioactive carbon in a plant or animal at death is known. Volcanic rock can also be used; its starting point is the moment when it cooled and crystallized.
There is a problem with these methods, however. Radioactive carbon decays so fast that it is useful only after about 40,000 years ago, and the radioactive potassium in volcanic rock decays so slowly that it is useful only before about 150,000 years ago. This leaves a huge gap of time not covered. Physicists are constantly developing other techniques. Perhaps the most promising of these is thermoluminescence, a way of measuring how long ago a rock like flint was heated. Since flint was often used for making tools, it frequently found its way into campfires, where it was heated enough to establish a starting point for thermoluminescence.
Thus, although geologists are beginning to understand the climatic framework of Ice Age chronology, and are beginning to flesh it out by associating particular sites with particular cold or warm spells, an enormous amount of work remains to be done.
Ice Age Climates and Ice Age People
The fastest great movement of the Ice Age glaciers probably averaged less than 10 miles in a lifetime. Ice Age people at best could have been only vaguely aware of the climatic transformations that seem so large in hindsight. For small tribes living on wild foods, with only the spoken word to link them to the past, such changes would probably be completely hidden by changes on a much smaller scale, fluctuations from year to year or from decade to decade.
We know a little about how Ice Age peoples adapted to their environment, although much of what we know is puzzling. The earliest inhabitants of Europe seem to have been confined primarily to more temperate environments, but by Middle Paleolithic times, Neanderthals (the immediate predecessors of modern humans in Europe) were also able to live in cold if not truly arctic condi tions. In warm environments, the animals they hunted were forest dwellers (red deer, fallow deer, wild pigs and cattle), while in harsher environments the animals were species that inhabited cold prairies or steppes (reindeer, horses, bison, and woolly mammoths). We have to remember that we are tropical animals. It is possible that Ice Age Europeans evolved some biological defenses against the cold that both we and our earlier African ancestors lacked (see the article by Nancy Minugh-Purvis, this issue), but it is doubtful that they could have lived even in temperate climates without fire, clothing, and shelter.
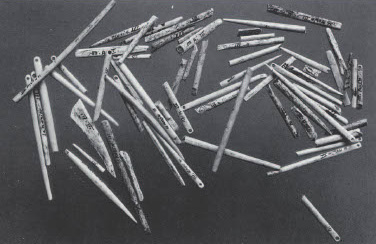
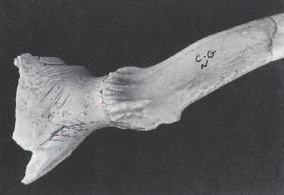
There is good evidence for the regular use of fire in Ice Age Europe. What is surprising, though, is that before the Upper Paleolithic, when fully modern humans appear in Europe, we have less evidence of either shelters or clothing than we might expect, given the cold climates people inhabited. There are a few stone pavements or stone “circles” that have been interpreted as shelters, but they are rare and usually controversial. By contrast, in the Upper Paleolithic, evidence of tents, paved floors, storage pits, and the like are both much more common and indubitably real. Likewise, in the Upper Paleolithic, we find the kinds of bone needles and awls we would expect if people were making clothes (Fig. 13). But in the Lower and Middle Paleolithic, we find only ambiguous clues that may point to the use of clothing: a few broken bones with polished tips that may have been used as awls, and in two sites in France, the foot bones of leopards or bears, sometimes with cut marks that may indicate that these animals were skinned (Fig. 14). It is hard to imagine that these ice Age people lived without clothing or shelter; but the lack of specialized tools indicates that their clothing may have been crude by the standard of more recent cold-climate hunters such as Eskimos, and the lack of traces of shelters implies that these, too, were crude and flimsy.
Because the Ice Age climate was so variable, archaeologists cannot speak in general terms about its impact. Even biological, evolutionary developments are hard to link to climatic change. This is not because the changes occurred too slowly, but because there were so many of them. One cannot explain an evolutionary development by a change in climate when the same change had already occurred many times with no biological effect.
In any case, it was in a varying climate and environment that the people described in this issue lived their lives. This is something we share with them, although we, like they, live our daily lives unaware of the larger cycles of change. The Ice Ages have in large measure shaped the world we know today, and we are indebted to them not only for spectacular mountain landscapes and 10,000 Minnesota lakes, hut also for the many species that evolved during them; reindeer, horses, polar bears—and Homo sapiens.